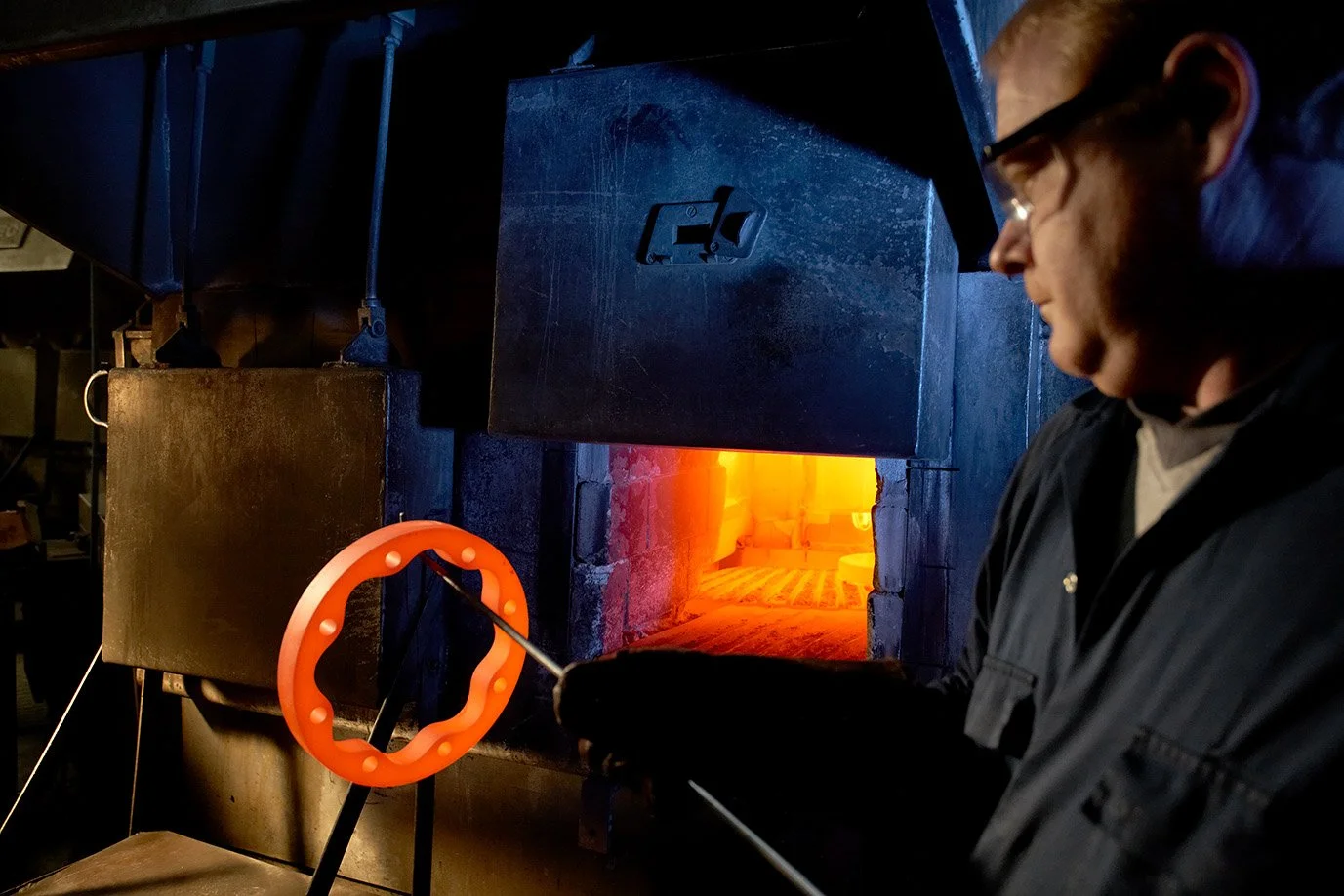
Heat Treatment
Imagine you're a piece of metal, tough but tired, and you need a day at the spa to get back to your best self. Welcome to the world of heat treatment, where metal gets pampered, rejuvenated, and strengthened through a series of special thermal treatments.
-
Carburising is a heat treatment process used to harden the surface of low-carbon steel or iron parts while maintaining a softer, tougher core. This is achieved by introducing carbon into the surface layer of the material at high temperatures. The process can significantly improve the wear resistance and fatigue strength of the component.
Here's a step-by-step explanation of the carburising process:
Preparation: The steel or iron parts to be carburised are cleaned to remove any surface contaminants such as oil, dirt, or rust.
Heating: The parts are heated in a furnace to a temperature typically between 850°C to 950°C (1562°F to 1742°F). This temperature range is above the critical temperature of steel, where the steel transforms into austenite, a phase that can absorb carbon.
Introduction of Carbon: At the carburising temperature, the parts are exposed to a carbon-rich environment. This can be done using various methods:
Gas Carburising: The parts are heated in a furnace with a carbon-containing gas, like methane or propane.
Liquid Carburising: The parts are immersed in a molten salt bath that contains carbon-bearing compounds.
Diffusion: The carbon atoms diffuse into the surface layer of the steel. The depth of the carbon penetration depends on the temperature and the duration of exposure. The longer the exposure and higher the temperature, the deeper the carbon will penetrate.
Quenching: After carburising, the parts are usually quenched in oil or water. This rapid cooling process transforms the carbon-rich surface layer into a hard, wear-resistant martensitic structure while retaining a softer, tougher core.
Tempering (Optional): To reduce brittleness and relieve internal stresses caused by quenching, the parts may undergo a tempering process. This involves reheating the parts to a lower temperature (typically between 150°C to 200°C) and then cooling them slowly.
Advantages of Carburising:
Increased surface hardness and wear resistance.
Improved fatigue strength due to a hardened surface layer.
The core remains tough and ductile, providing overall toughness.
Applications:
Automotive components such as gears, crankshafts, and camshafts.
Bearings and other machine parts subjected to high wear and fatigue.
Tools and dies that require a hard surface and a tough core.
Carburising is widely used in industries where the combination of surface hardness and core toughness is essential for the performance and longevity of mechanical components.
-
Hardening and tempering are heat treatment processes used to enhance the properties of steel, making it both hard and tough. These processes are often performed in sequence to achieve the desired mechanical properties in a metal component.
Hardening
Heating: The steel is heated to a temperature above its critical point, usually between 800°C to 900°C (1472°F to 1652°F), depending on the specific type of steel. At this temperature, the steel transforms into austenite, a phase that can dissolve more carbon than ferrite or pearlite.
Soaking: The steel is held at the austenitizing temperature for a period, allowing the carbon to distribute uniformly within the austenite.
Quenching: The steel is rapidly cooled, typically in water, oil, or another cooling medium. This rapid cooling prevents the carbon atoms from diffusing out of the austenite, resulting in the formation of martensite, a very hard and brittle microstructure.
Effects of Hardening:
Increased Hardness: The formation of martensite significantly increases the hardness of the steel.
Increased Brittleness: The high hardness comes with increased brittleness, making the steel more prone to cracking and fracture.
Tempering
Tempering is performed after hardening to reduce the brittleness of the martensitic steel and improve its toughness.
Reheating: The hardened steel is reheated to a temperature below the critical point, typically between 150°C to 700°C (302°F to 1292°F). The exact temperature depends on the desired balance between hardness and toughness.
Holding: The steel is held at the tempering temperature for a specific duration, allowing for the transformation of the microstructure.
Cooling: The steel is then slowly cooled, usually in air.
Effects of Tempering:
Reduced Brittleness: Tempering reduces internal stresses and brittleness, making the steel tougher and more ductile.
Controlled Hardness: The hardness of the steel decreases slightly, but it remains much harder than its pre-hardened state.
Improved Toughness: The steel becomes more resistant to impact and mechanical stress.
Applications
Hardening and tempering are used for components that require a combination of high strength, wear resistance, and toughness, such as:
Cutting tools and blades
Automotive parts like gears and shafts
Springs
Structural components in machinery
Summary
Hardening involves heating steel above its critical temperature, soaking it to distribute carbon, and then quenching it to form martensite, resulting in a hard but brittle structure.
Tempering involves reheating the hardened steel to a lower temperature, holding it, and then cooling it to reduce brittleness and increase toughness while maintaining significant hardness.
By carefully controlling the temperatures and durations of these processes, manufacturers can tailor the mechanical properties of steel to suit specific applications.
-
Press quenching is a specialised heat treatment process used primarily for complex or precision-shaped components, where controlling distortion and maintaining dimensional accuracy are critical. It combines the principles of quenching with the application of mechanical force to ensure that the part retains its desired shape during the cooling process. This method is particularly useful for components that are prone to warping or distortion when cooled rapidly.
Steps in Press Quenching
Heating: The component is first heated to its austenitizing temperature, typically in the range of 800°C to 900°C (1472°F to 1652°F), depending on the type of steel. At this temperature, the steel transforms into austenite, a phase that can dissolve more carbon than other phases like ferrite or pearlite.
Transfer to Quenching Press: The heated component is quickly transferred to a quenching press. This transfer must be done swiftly to avoid any significant loss of temperature.
Positioning and Clamping: The component is positioned within the quenching press, where it is clamped securely. The press is designed to hold the part in the exact shape and dimensions required. Clamping ensures that the part does not warp or distort during quenching.
Quenching: Quenching fluid, usually oil or water, is introduced to rapidly cool the component. The press maintains mechanical pressure on the component throughout the quenching process, ensuring that the part retains its desired shape as it transforms from austenite to martensite. The cooling rate is controlled to balance hardness and minimize internal stresses.
Releasing and Inspection: After the component has cooled sufficiently and transformed into martensite, it is removed from the press. The part is then inspected for dimensional accuracy and any potential distortions or defects.
Advantages of Press Quenching
Dimensional Accuracy: The primary advantage of press quenching is the ability to maintain tight dimensional tolerances and complex shapes with minimal distortion.
Reduced Warping and Cracking: By applying mechanical pressure during quenching, the process reduces the risk of warping, cracking, and other forms of distortion that can occur in traditional quenching methods.
Improved Mechanical Properties: Press quenching can improve the mechanical properties of the component by ensuring a uniform transformation to martensite, resulting in consistent hardness and strength.
Applications
Press quenching is used for components that require precise dimensional control and high mechanical performance, such as:
Gears and gear shafts
Precision tools and dies
Bearing races
Automotive parts like transmission components
Aerospace components
Summary
Press quenching is a heat treatment process that combines rapid cooling with mechanical pressure to control the shape and dimensions of complex or precision components. By maintaining the desired geometry during quenching, this method minimizes distortion and ensures high-quality mechanical properties, making it ideal for critical applications in various industries.
-
Stress relieving is a heat treatment process used to reduce residual stresses in materials, particularly metals, without significantly altering their structure or mechanical properties. Residual stresses are internal stresses that remain in a material after it has been manufactured or processed, such as through welding, casting, machining, or forming. These stresses can lead to distortion, cracking, or premature failure of the material if not properly managed.
Steps in Stress Relieving
Heating: The material is uniformly heated to a specific temperature, typically below its critical temperature. For most steels, this temperature range is between 550°C to 650°C (1022°F to 1202°F). For other materials like aluminum, the stress relieving temperature would be lower.
Soaking: The material is held at the stress relieving temperature for a sufficient amount of time, which allows the residual stresses to be redistributed and reduced. The soaking time depends on the material thickness and the extent of the residual stresses but usually ranges from 30 minutes to several hours.
Cooling: The material is then slowly cooled to room temperature. Slow cooling is crucial to avoid the introduction of new stresses that can occur with rapid cooling.
Advantages of Stress Relieving
Reduced Risk of Distortion and Cracking: By reducing residual stresses, stress relieving minimizes the risk of distortion or cracking during subsequent processing or service.
Improved Dimensional Stability: Components that have undergone stress relieving are more dimensionally stable, meaning they are less likely to deform over time or under load.
Enhanced Mechanical Properties: While the primary goal of stress relieving is not to change the material's mechanical properties, it can indirectly improve performance by eliminating stress concentrations that can act as sites for crack initiation.
Applications
Stress relieving is commonly applied to a wide range of components across various industries, including:
Welded Structures: To reduce stresses introduced during the welding process and prevent weld cracking.
Machined Parts: To relieve stresses generated during machining, particularly for components requiring high precision.
Castings and Forgings: To reduce stresses formed during solidification and cooling.
Pressure Vessels and Pipelines: To ensure structural integrity and prevent stress corrosion cracking.
Summary
Stress relieving is a heat treatment process aimed at reducing residual stresses in materials without significantly altering their overall structure or mechanical properties. The process involves controlled heating, soaking, and slow cooling of the material. By reducing internal stresses, stress relieving enhances the dimensional stability and structural integrity of components, making it a crucial step in the manufacturing and maintenance of various metal parts and structures.
-
Annealing is a heat treatment process used to alter the physical and sometimes chemical properties of a material, primarily metals, to increase its ductility, reduce its hardness, and relieve internal stresses. The process involves heating the material to a specific temperature, maintaining that temperature for a period, and then cooling it at a controlled rate. Annealing is widely used in metalworking to make materials easier to work with and to enhance their mechanical properties.
Steps in the Annealing Process
1. Heating: The material is slowly heated to a specific temperature, typically within a range where recrystallization can occur. For steel, this temperature is usually between 700°C to 900°C (1292°F to 1652°F). The exact temperature depends on the type of metal and the desired properties.
2. Soaking: The material is held at the annealing temperature for a period sufficient to allow the internal structure to fully transform. This soaking time allows for the formation and growth of new, strain-free grains within the metal. The soaking time depends on the material's thickness and composition but generally ranges from minutes to hours.
3. Cooling: The material is then cooled at a controlled rate. The cooling rate can vary depending on the desired properties:
- Full Annealing: Involves slow cooling in the furnace, often at a rate of 20°C to 50°C (36°F to 90°F) per hour. This produces a coarse pearlite structure in steel, which is soft and ductile.
- Process Annealing: Involves faster cooling, typically in air, which can be used for less critical applications where maximum ductility is not required.
- Spheroidizing Annealing: Used for high-carbon steels, involves holding the steel at a temperature just below the critical point for an extended period and then cooling it slowly. This produces a spheroidized structure that enhances machinability.
Types of Annealing
1. Full Annealing: Aimed at achieving maximum ductility and reducing hardness to the lowest possible level for the given alloy. Commonly used for low and medium carbon steels.
2. Process Annealing: Often used in the middle of a production process to restore ductility lost during work hardening. It involves heating the material to a lower temperature than full annealing.
3. Stress-Relief Annealing: Used to relieve residual stresses without changing the material's microstructure significantly. The temperature is lower than that used for full or process annealing.
4. Spheroidizing Annealing: Used to produce a globular form of carbide in steel, which improves machinability. It involves prolonged heating at a temperature just below the critical point.
Advantages of Annealing
- Improved Ductility: The material becomes softer and more ductile, making it easier to form, bend, and machine.
- Reduced Hardness: The hardness of the material is reduced, which is beneficial for subsequent processing steps.
- Relieved Internal Stresses: Residual stresses from previous manufacturing processes are minimized, reducing the risk of distortion and cracking.
- Enhanced Mechanical Properties: The material’s toughness and workability are improved, making it more suitable for various applications.
Applications
Annealing is widely used across various industries for numerous applications, including:
- Manufacturing: To improve the workability of metals for further processing.
- Automotive: To enhance the ductility and toughness of components like gears and shafts.
- Construction: To ensure the structural integrity of steel beams and other construction materials.
- Toolmaking: To improve the machinability of tool steels and extend the life of cutting tools.
Summary
Annealing is a crucial heat treatment process that enhances the ductility, reduces the hardness, and relieves internal stresses in metals. By carefully controlling the heating, soaking, and cooling stages, manufacturers can tailor the mechanical properties of metals to suit specific applications, making them easier to work with and more durable in their final use.
-
Normalising is a heat treatment process applied to ferrous metals, such as steel, to refine their grain structure, enhance mechanical properties, and improve machinability. It involves heating the metal to a temperature above its critical range, holding it at that temperature to achieve uniformity, and then allowing it to cool in still air. Normalising is often used to relieve internal stresses, homogenize the microstructure, and prepare the metal for further processing.
Steps in the Normalising Process
1. Heating: The metal is uniformly heated to a temperature typically between 800°C and 950°C (1472°F to 1742°F), which is above its critical transformation range. For steel, this temperature range is chosen to transform the microstructure into austenite.
2. Soaking: The metal is held at the normalising temperature for a sufficient time to ensure that the entire part reaches uniform temperature and the desired microstructural changes occur. The soaking time depends on the size and thickness of the part but generally ranges from a few minutes to several hours.
3. Cooling: The metal is removed from the heat source and allowed to cool in still air. This cooling rate is slower than quenching but faster than annealing, leading to the formation of a refined and uniform microstructure, usually pearlite or ferrite, depending on the alloy composition.
Objectives and Benefits of Normalising
- Grain Refinement: Normalising produces a finer and more uniform grain structure, which improves the mechanical properties of the metal, such as toughness and strength.
- Homogenisation: It helps to eliminate chemical segregation and homogenize the composition, making the metal's properties more uniform throughout the part.
- Stress Relief: The process relieves internal stresses that may have developed during previous processing steps, such as forging, casting, or welding.
- Enhanced Machinability: The refined grain structure and improved uniformity enhance the machinability of the metal, making it easier to cut, shape, and form.
- Improved Ductility: Normalising can increase the ductility of the metal, making it more pliable and less prone to cracking or brittle failure.
Applications
Normalising is widely used in various industries for components that require improved mechanical properties and dimensional stability:
- Automotive: Gears, shafts, axles, and other critical components that require uniform properties and enhanced strength.
- Aerospace: Structural components, landing gear, and other parts subjected to high stresses and requiring high toughness.
- Construction: Beams, columns, and other structural elements that need to withstand load-bearing applications.
- Manufacturing: Tools, dies, and machine parts that require precise machining and dimensional stability.
- Oil and Gas: Pipelines, valves, and other components that need to resist high pressures and harsh environments.
Summary
Normalising is a heat treatment process that refines the grain structure, enhances mechanical properties, and improves the uniformity of ferrous metals. By heating the metal above its critical range, soaking it, and then allowing it to cool in still air, normalising relieves internal stresses, improves machinability, and increases ductility. Widely used in automotive, aerospace, construction, and manufacturing industries, normalising ensures that metal components have the necessary properties to perform reliably in demanding applications.
Capacities
-
3 furnaces - 760mm (w) x 1220mm (d) x 760mm (h)
-
6 furnaces - 1066mm (dia) x 914mm (h)
-
2 furnaces - 1000mm (w) x 1000mm (d) x 1000mm (h)
1 furnace - 760mm (w) x 12200mm (d) x 760 (h)
-
3 furnaces - 368mm (dia) x 305mm (h)
-
3 presses - 406mm (dia) x 203mm (h)